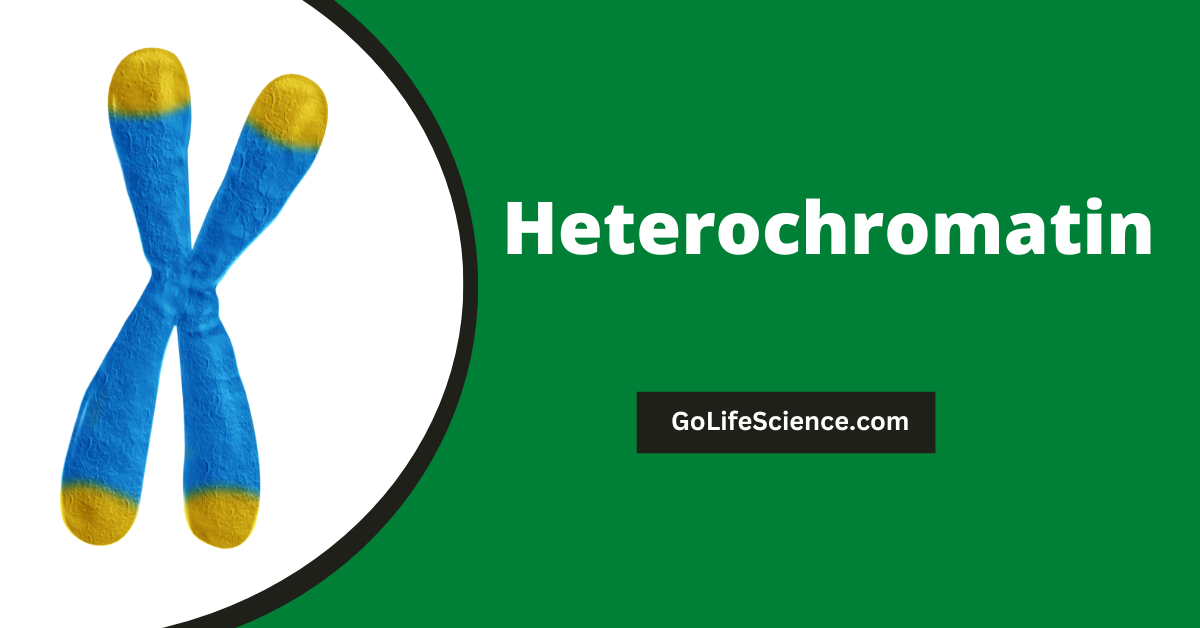
Heterochromatin is a form of chromatin that is very densely packed and transcriptionally inactive. While DNA in euchromatin regions is loosely packed and accessible to the transcription machinery, allowing active gene expression, Heterochromatin keeps the underlying DNA inaccessible and silenced.
This heterochromatic state plays essential roles in regulating gene expression, maintaining genome stability, and establishing cell identity.
What is Heterochromatin?
Heterochromatin consists of large regions of highly condensed, transcriptionally inactive DNA found mainly near centromeres and telomeres. In contrast to loosely packed euchromatin, where genes are actively transcribed, Heterochromatin is characterized by tight chromatin packaging that renders genes inaccessible. This compaction is achieved through interactions between DNA and histone proteins.
Histones act as spools that DNA winds around, and the binding of DNA to histones facilitates packing into a condensed structure. Histone proteins undergo chemical modifications, such as methylation and acetylation, that influence the compaction state of chromatin.
Heterochromatin is defined by the presence of histone modifications associated with gene silencing, such as methylation of histone H3 lysine 9 (H3K9me). These types of repressive histone marks lead to the formation of condensed, untranscribed chromatin.
The primary sequence of heterochromatic DNA consists mostly of repetitive elements that do not code for proteins. This includes large arrays of satellite repeats as well as an abundance of transposable elements. Non-coding RNA transcripts derived from repetitive regions also contribute to the structure of Heterochromatin.
Functions of Heterochromatin
In 1928, based on histologist observations, Emil HEITZ defined Heterochromatin (HC) as being the chromosomal segments that appear extremely condensed and dark in color in the interphase nucleus.
In fact, chromatin consists of a tangle of fibers, the diameter of which not only varies during the cell cycle but also depends on the region of the chromosome observed.
Although it does not contain actively expressed genes, Heterochromatin carries out a number of essential duties in the genome:
- Gene silencing: The condensed structure of Heterochromatin causes the repression of genes within and surrounding heterochromatic regions. By silencing repetitive elements, Heterochromatin prevents spurious transcription from these DNA repeats.
- Genome organization: Heterochromatin helps establish higher order chromatin structure and nuclear architecture. It clusters near the nuclear periphery and organizes chromosome territories.
- Chromosome segregation: At centromeres plays a key role in the proper separation of chromosomes during cell division. Condensation of pericentric Heterochromatin enables centromere function.
- Genome stability: Repetitive elements like transposons can cause double-strand DNA breaks and mutations if expressed. It suppresses these harmful repetitive elements to maintain genome integrity.
- Epigenetic inheritance: It states can be inherited through cell divisions. Daughter cells will maintain silencing of heterochromatic regions present in the parent cell.
By compacting DNA repeats and repressing nearby genes, Heterochromatin enables proper regulation of the genome during development and prevents genomic instability.
Types of Heterochromatin
Not all Heterochromatin is identical. There are two main varieties that differ in their distribution across cell types and developmental stages:
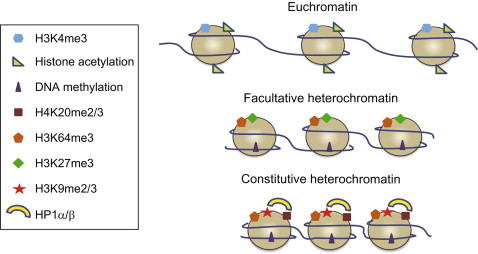
A. Constitutive Heterochromatin
- Constitutive HC is always compacted and genetically inactive in all cell types. It is found concentrated around the centromeres and telomeres of all chromosomes.
- Constitutive HC performs important cellular duties such as chromosome segregation, and therefore must remain condensed in every cell.
The main components of constitutive Heterochromatin are satellite repeats and transposable elements. Certain families of repetitive DNA are specifically enriched in pericentric or telomeric regions. Constitutive Heterochromatin is characterized by high levels of H3K9 methylation.
B. Facultative Heterochromatin
Facultative Heterochromatin encompasses regions that can shift between active and inactive transcriptional states in diverse cell types or during various developmental stages. It serves to silence genes that are not appropriate for a particular lineage while keeping tissue-specific genes ready for activation.
For example, during mammalian development, This is established on one of the two X chromosomes in females to accomplish X-chromosome inactivation and dosage compensation. Specific sets of genes are silenced by facultative HC in different cell lineages.
The composition of facultative HC can include protein-coding genes, transposons, and tandem repeats. Histone modifications are also variable, with enrichment in H3K27me3 or H2A ubiquitination in certain contexts. The flexible nature of facultative Heterochromatin allows cell-specific control of gene expression.
Formation and Maintenance of Heterochromatin

Heterochromatin establishment and propagation involves coordinated action of DNA methylation, histone modifications, chromatin proteins, and RNA transcripts.
A. Histone Methyltransferases
SUV39H1 kickstarts Heterochromatin formation by placing H3K9me3 marks, which recruit HP1. This HP1 then attracts more SUV39H1 to spread the heterochromatic state.
EHMT enzymes can also methylated H3K9 as well as H4K20 for added silencing. The Polycomb complex deposits H3K27me3 for facultative Heterochromatin formation.
B. Histone Deacetylases
HDACs like SIRT1 remove acetyl groups from histone tails, increasing positive charge and chromatin compaction. This complements the action of repressive histone methylation.
C. DNA Methylation
DNA methyltransferases like DNMT3A/B establish CpG methylation at repetitive elements. This reinforces the silent state and prevents deleterious activation of transposons.
D. Non-coding RNAs
Transcription of satellite repeats or transposons can produce siRNAs that target complementary sequences for silencing, helping reinforce Heterochromatin. The RNAi pathway utilizes these small RNAs.
Additionally, certain long non-coding RNAs directly recruit chromatin modifiers like PRC2 to assist in Heterochromatin nucleation.
Through the joint activity of these various factors, Heterochromatin structure can be established and epigenetically maintained through generations.
Functions of Heterochromatin by Genomic Region
The location of Heterochromatin within the genome determines its specialized functions. The main functional regions are centromeric, telomeric, and interspersed.
A. Pericentric Heterochromatin
The Heterochromatin surrounding the centromeres, consisting largely of satellite repeat DNA, carries out essential duties for chromosome dynamics.
- Cohesion: Enables sister chromatids to stick together after replication until separation.
- Kinetochore assembly: The kinetochore forms on centromeric hc to attach to spindle fibers.
- Chromosome segregation: Condensation of pericentric Heterochromatin facilitates the pulling apart of sisters during cell division.
- Structural integrity: Repeats help hold two sister chromatids together through multiple rounds of cell division.
Pericentric Heterochromatin enables proper chromosome inheritance.
B. Telomeric Heterochromatin
The repetitive DNA at chromosome ends forms protective caps that preserve genome stability:
- Prevents fusion: Stops chromosomes from mistakenly joining to other broken ends.
- Blocks degradation: Stops nucleases from degrading the chromosome end.
- Replication: Telomeric repeats maintain the telomerase template for replication.
- Protein recruitment: Special proteins bind telomeric repeats to facilitate end protection.
Telomeric Heterochromatin performs essential capping duties.
C. Interstitial Heterochromatin
Scattered heterochromatic regions within chromosome arms regulate genes and repetitive elements:
- Developmental genes: Silences lineage-inappropriate developmental regulators via facultative Heterochromatin. Enables cell differentiation.
- Transposons: Prevents transposon activation and insertion mutations which can destabilize the genome.
- Gene clusters: Can silence entire local neighborhoods to regulate coordinated expression of gene clusters.
The location of Heterochromatin within centromeres, telomeres, or chromosome arms determines its specialized roles in chromosome dynamics, genome stability, development, and defense against selfish genetic elements.
Importance of Heterochromatin in Genome Function
Although lacking in protein-coding genes, Heterochromatin performs indispensable functions that impact genetic stability, cell identity, and overall genome regulation.
A. Suppression of Transposons
Transposons are mobile DNA elements that can copy and insert themselves disruptively within genomes. Heterochromatin silences these parasitic elements through compacted chromatin and DNA methylation. This prevents transposon-induced mutations that would destabilize the genome.
B. Regulation of Gene Expression
In addition to silencing nearby genes, Heterochromatin also indirectly regulates gene transcription by organizing the 3D structure of the genome within the nucleus. Chromatin interactions partition the genome into active and inactive compartments.
C. Stabilization of Repetitive Regions
The highly repetitive DNA within Heterochromatin is prone to inappropriate homologous recombination. By compacting heterochromatic regions, the cell prevents deleterious rearrangements between repeats that would jeopardize genome integrity.
D. Epigenetic Inheritance
The heterochromatic state, characterized by histone modifications and DNA methylation, can be transmitted to daughter cells to maintain silencing of repetitive elements and regulation of gene expression. This epigenetic inheritance of Heterochromatin is crucial for cellular identity.
E. Developmental Gene Regulation
Facultative Heterochromatin established during differentiation is key for silencing developmental genes not needed in a particular cell lineage. This provides stability to cell identity and prevents dysregulated growth such as cancer.
F. Chromosome Dynamics
The constitutive pericentric Heterochromatin enables proper chromosome segregation, cohesion, and kinetochore function. Without Heterochromatin, chromosomes would fail to separate correctly during cell division.
Clearly Heterochromatin does more than merely take up space within the genome. This silent chromatin safeguards genome stability, sculpts the epigenome, and enables the cell to dynamically regulate gene expression while preserving its core identity. Heterochromatin powers and protects the genome.
Heterochromatin diseases
Heterochromatin diseases are a group of genetic disorders that involve abnormalities in the structure or function of Heterochromatin, a tightly packed form of DNA found in the cell nucleus. Heterochromatin plays a crucial role in regulating gene expression and maintaining genome stability. When there are defects or mutations in the genes associated with Heterochromatin, it can lead to various diseases and health conditions. Here are a few examples of Heterochromatin-related diseases:
- Facioscapulohumeral Muscular Dystrophy (FSHD): FSHD is a genetic muscle disorder that is linked to changes in Heterochromatin structure. It is primarily caused by the deletion of repetitive DNA sequences near the DUX4 gene, which leads to changes in Heterochromatin organization and the inappropriate activation of certain genes in muscle cells.
- ICF Syndrome (Immunodeficiency, Centromere Instability, and Facial Anomalies Syndrome): ICF syndrome is a rare immunodeficiency disorder characterized by recurrent infections, instability of centromeric Heterochromatin, and distinctive facial features. Mutations in genes like DNMT3B, which is involved in DNA methylation and Heterochromatin formation, can cause this syndrome.
- Rett Syndrome: Rett syndrome is a neurodevelopmental disorder predominantly seen in females. It is associated with mutations in the MECP2 gene, which codes for a protein that interacts with Heterochromatin and is involved in regulating gene expression. These mutations disrupt normal Heterochromatin function and lead to neurological and developmental problems.
- Fragile X Syndrome: Fragile X syndrome is another neurodevelopmental disorder, and it is caused by a mutation in the FMR1 gene. The FMR1 protein is involved in RNA transport and translation regulation. Dysregulation of Heterochromatin and gene expression in the vicinity of the FMR1 gene contributes to the cognitive and behavioral symptoms of this syndrome.
- Aging-related Diseases: Heterochromatin undergoes changes during the aging process, and these changes have been implicated in various age-related diseases such as cancer, neurodegenerative disorders, and cardiovascular diseases. Dysfunctional Heterochromatin can lead to altered gene expression patterns that contribute to the pathogenesis of these diseases.
Understanding the role of Heterochromatin in these diseases is essential for developing potential therapeutic strategies and gaining insights into the molecular mechanisms underlying these conditions. Research in this field continues to expand our knowledge of how Heterochromatin contributes to both health and disease.
Conclusion
In summary, our journey into the realm of Heterochromatin has unveiled its intricate structure, multifaceted functions, and pivotal role in the realm of genetics.
As we continue to delve deeper into the mysteries of this chromatin type, we gain valuable insights into the regulation of gene expression, development, and the intricate dance of genetic information within our cells.
Heterochromatin, with its ability to silence or activate genes in a dynamic manner, serves as a fascinating area of study with far-reaching implications for our understanding of genetics and its implications for health and disease.