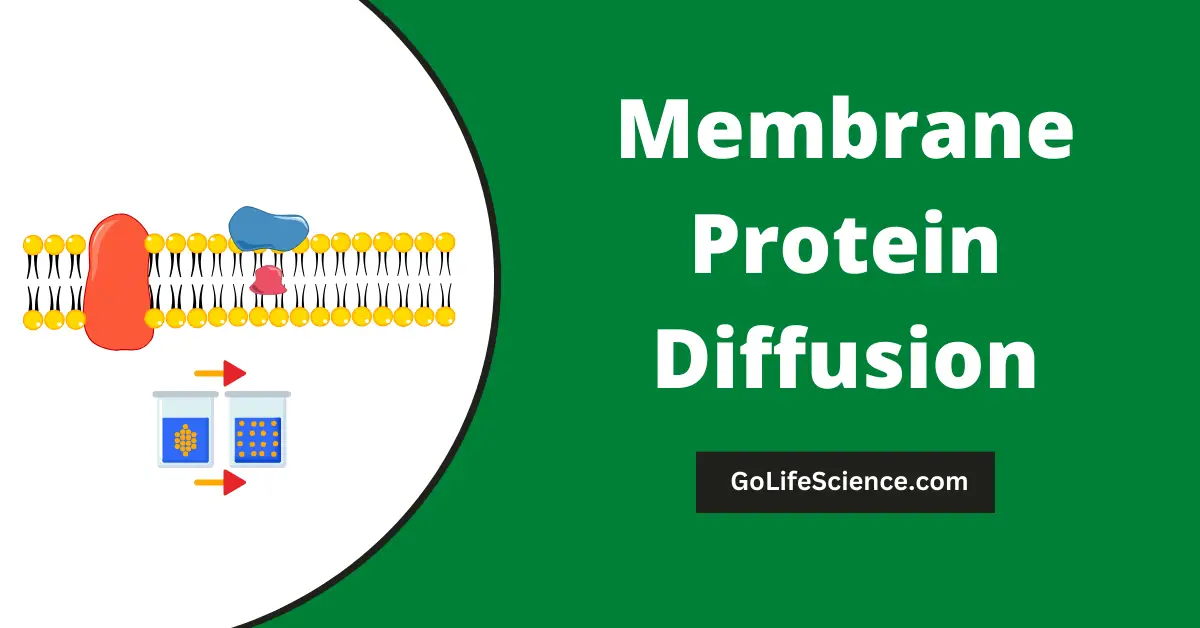
Membrane protein diffusion refers to the movement of membrane proteins laterally or transversely within the lipid bilayer of biological membranes.
This process is critical in many cellular activities and allows membrane proteins to localize, assemble, and interact with other components properly.
Understanding the dynamics and mechanisms of membrane protein diffusion provides important insights into protein function, cell signaling, membrane organization, and disease pathogenesis.
Importance of Studying Membrane Protein Diffusion:
Membrane protein diffusion is essential for:
- Cell signaling: enables receptor proteins to interact with signaling molecules and propagate signals into the cell interior.
- Membrane transport: allows transport proteins to migrate to optimal positions for translocation of ions and molecules.
- Membrane domain organization: contributes to the formation and maintenance of specialized membrane microdomains.
- Adaptation: allows membrane protein redistribution in response to environmental changes.
- Disease mechanisms: aberrant membrane protein diffusion can contribute to disorders like cystic fibrosis, Alzheimer’s, and more.
Overall, comprehending the nuances of membrane protein mobility and diffusion mechanisms provides a deeper understanding of dynamic biological membrane structure and critical cellular activities.
Table of Contents
Types of Membrane Proteins
Two major classes of membrane proteins undergo diffusion:
Integral Membrane Proteins
- Embedded within the membrane lipid bilayer.
- Usually, transmembrane proteins that span the membrane.
- Mediate cell signaling, adhesion, and transport of molecules.
- Examples: receptor proteins, ion channels, transporters.
Peripheral Membrane Proteins
- Associated with membrane surface but do not penetrate bilayer.
- Attach via lipid anchors, electrostatic interactions, and other proteins.
- Play key roles in cell structure, trafficking, and signaling.
- Examples: cytoskeletal proteins signaling enzymes.
Both types diffuse laterally and transversely, but integral proteins are more restricted due to tight membrane association.
Modes of Membrane Protein Diffusion
Membrane protein diffusion can occur through various modes characterized by different behaviors and mechanisms. The primary modes of membrane protein diffusion include:
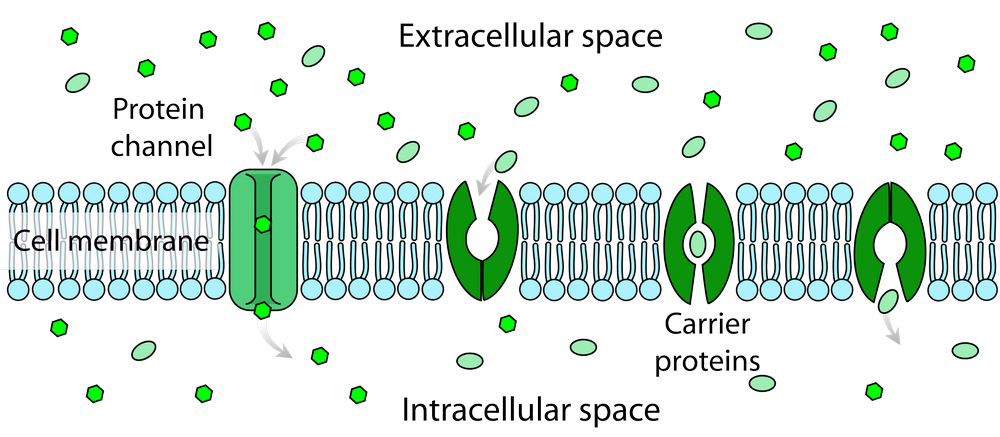
1. Free Diffusion: In this mode, membrane proteins move randomly within the lipid bilayer, primarily driven by thermal motion. These proteins can diffuse laterally and rotate freely without any specific constraints. Free diffusion is typical for small, relatively mobile membrane proteins.
2. Hindered Diffusion: Some membrane proteins experience hindered diffusion due to interactions with other molecules, such as membrane lipids, cytoskeletal elements, or other proteins. This mode of diffusion results in slower and more restricted movement than free diffusion.
3. Directed Diffusion: Certain membrane proteins exhibit directed diffusion, where specific cues or interactions guide their movement. For instance, membrane receptors may move along a gradient towards their ligands, while motor proteins like kinesin and dynein facilitate cargo transport within the cell.
4. Confined Diffusion: Membrane proteins can be confined to specific microdomains or structures within the membrane, limiting their lateral movement. This confinement can be achieved through interactions with other proteins, such as anchoring or clustering within membrane rafts.
5. Corralled Diffusion: In this mode, membrane proteins move within defined corrals or barriers formed by the cytoskeleton or membrane components. They can hop between these corrals, resulting in restricted lateral diffusion.
6. Anomalous Diffusion: Some membrane proteins exhibit anomalous diffusion, where their movement does not follow classical Brownian motion. This behavior is characterized by subdiffusion (slower than expected), superdiffusion (faster than expected), or other non-linear motion patterns. Anomalous diffusion is often attributed to the complex and dynamic organization of the membrane environment.
The specific mode of diffusion for a membrane protein depends on its interactions with the surrounding environment, its size, the presence of binding partners, and the organization of the membrane itself. The interplay of these factors determines how membrane proteins move within the lipid bilayer and play essential roles in cellular processes.
Membrane proteins primarily exhibit two types of diffusion:
Lateral Diffusion
- Movement within the plane of the membrane.
- Allows mobility to interact with partners and assemble domains.
- Rate determined by size, interactions, crowding, and membrane viscosity.
The Lateral diffusion of a membrane protein is present within the phospholipid bilayer.
Transverse Diffusion (Flip-Flop)
- Movement from one bilayer leaflet to the other.
- Allows exposure of distinct protein regions.
- Slow process due to high energy barrier for protein reorientation.
Transverse diffusion of a membrane protein between bilayer leaflets.
Factors Affecting Membrane Protein Diffusion
Here are some key factors that affect membrane protein diffusion:
a. Lipid environment
The lipid composition of the membrane bilayer influences membrane protein diffusion. More saturated lipids tend to restrict diffusion, while unsaturated lipids increase fluidity and diffusion rates. Cholesterol also affects membrane fluidity.
- Lipid type impacts membrane fluidity and viscosity.
- Cholesterol and saturated lipids decrease fluidity.
- Polyunsaturated lipids increase fluidity.
b. Protein size and shape
Larger membrane proteins and proteins with more transmembrane domains tend to diffuse more slowly. The shape of the protein’s hydrophobic regions about the membrane lipids also impacts diffusion.
c. Crowding and obstacles
A high concentration of other proteins or barriers in the membrane can hinder the diffusion of membrane proteins by creating steric hindrances.
d. Protein-protein interactions
Membrane proteins, cytoskeletal components, and extracellular matrix can bind to each other. These interactions can reduce their lateral mobility.
- Direct interactions impede diffusion.
- Aggregation into complexes or domains slows mobility.
e. Membrane thickness
The lipid bilayer’s hydrophobic thickness must match the protein’s hydrophobic surface. The mismatch can affect protein positioning and diffusion.
- More fluid membranes enable faster diffusion.
- Gel-phase membranes inhibit mobility.
f. Membrane compartmentalization
Some membranes have specialized lipid raft domains that separate proteins and affect mobility. The cytoskeleton can also corral membrane proteins.
g. Post-translational modifications
Modifications like palmitoylation can anchor proteins and restrict diffusion. Phosphorylation can also alter interactions.
h. Temperature
Increased temperature enhances membrane fluidity and protein diffusion rates. Cooler temperatures make the membrane more rigid and slow diffusion.
- Higher temperature enhances membrane fluidity.
- It leads to faster lateral and transverse diffusion.
The key factors are lipid environment, protein size/shape, Crowding, interactions, membrane dimensions, compartments, protein modifications, and temperature. All of these can alter the viscosity and protein constraints in the membrane.
Techniques to Study Membrane Protein Diffusion
Researchers utilize various techniques to analyze diffusion dynamics:
Fluorescence Recovery After Photobleaching (FRAP)
Fluorescence Recovery After Photobleaching (FRAP) is a powerful and widely used experimental technique in cell biology and biophysics. In FRAP, a specific region within a fluorescently labeled cell or sample is photobleached, causing the fluorescence in that area to vanish. By monitoring the subsequent fluorescence recovery over time, researchers can gain insights into the dynamics of molecular mobility and interactions within living cells. FRAP provides a means to study processes such as protein diffusion, membrane dynamics, and intracellular transport, offering valuable information about cellular dynamics and organization.
- Fluorescently labeled target protein, photobleach part of the sample.
- Monitor fluorescence recovery as unbleached proteins diffuse into the bleached area.
- Determine diffusion coefficient and mobile fraction.
Single-Particle Tracking (SPT)
Single-Particle Tracking (SPT) is a cutting-edge microscopy technique that enables the real-time monitoring of individual fluorescently labeled particles, such as molecules or nanoparticles, within biological systems or materials. By precisely tracking the positions and trajectories of single particles, SPT provides a granular understanding of their movement, diffusion, and interactions at the nanoscale.
This technique has revolutionized the study of dynamic processes in cell biology, neuroscience, and materials science, shedding light on phenomena like protein transport, receptor binding, and nanoparticle behavior. SPT’s ability to reveal heterogeneity and intricacies in particle dynamics has made it an invaluable tool for unraveling complex biological and material-related questions.
- Label individual proteins with quantum dots or gold nanoparticles.
- Track diffusion paths over time with high-resolution light microscopy.
- Reveal heterogeneity in diffusion behaviors.
Super-Resolution Microscopy
Super-resolution microscopy represents a groundbreaking advancement in optical imaging, surpassing the traditional diffraction limit and allowing scientists to visualize biological structures and nanoscale details with unprecedented precision. By overcoming the limitations that hindered conventional light microscopy, super-resolution techniques like STED, PALM, and SIM enable the visualization of cellular and subcellular structures at resolutions previously thought impossible.
This technology has profoundly impacted various scientific disciplines, from cell biology to materials science, offering a deeper understanding of intricate cellular processes, the architecture of nanomaterials, and the dynamics of molecular interactions. Super-resolution microscopy has become an indispensable tool for researchers, ushering in a new era of high-resolution imaging that has transformed our comprehension of the microcosmic world.
- Methods like STED and PALM offer nanoscale resolution.
- Visualize protein localization and interactions at the molecular scale.
- Provide details on membrane domains and dynamic distributions.
These and other approaches provide new insights into membrane protein mobility and localization.
Biological Significance of Membrane Protein Diffusion
The diffusion of membrane proteins in cell membranes has several important biological implications:
a. Cell signaling
Many membrane proteins, like receptors, must diffuse in the membrane to interact with other proteins and transduce signals. They have restricted diffusion limits signaling.
- Allows receptors to diffuse and interact with extracellular signals.
- It propagates downstream signaling via protein associations.
- Disruption can block signals and cause cellular dysfunction.
b. Transport
Channel and transporter proteins must diffuse to transport molecules across the membrane. Diffusion allows them to migrate to where they are needed.
- Allows transporters/channels to migrate to optimal positions.
- It is important for the exchange of ions, nutrients, and waste.
- Defects lead to transport deficiencies.
c. Cell mobility
Glycoproteins diffusing along the cell surface allow cells to move. Slowed diffusion inhibits cell motility.
d. Membrane maintenance
Lipids and proteins must diffuse laterally for membrane renewal and homeostasis. Lack of diffusion prevents membrane remodeling.
e. Cell polarization
Redistribution of diffusing proteins helps establish membrane asymmetry and polarity in processes like cell division. Limited diffusion reduces polarization.
f. Protein sorting
Sorting membrane proteins to various compartments involves diffusion to target membrane areas. Impeded diffusion causes sorting defects.
g. Enzyme regulation
Enzyme activity can rely on diffusion-limited encounters with substrates. Altered diffusion changes reaction kinetics.
h. Cell interactions
Surface proteins must diffuse to points of cell-cell contact like immunological synapses. Reduced mobility hinders cell interactions.
i. Organelle contacts
Mitochondria, ER, etc, interact at membrane contact sites. Diffusion allows proteins to reach these junctions.
Membrane protein diffusion impacts many cellular processes by enabling protein interactions, transport, signaling, mobility, organization, and interactions. It is a fundamental biophysical process in membrane biology.
Pathological Implications of Aberrant Membrane Protein Diffusion
Aberrant diffusion or improper localization of membrane proteins can contribute to many pathological conditions:
1. Cancer
Faulty diffusion of receptors like EGFR can lead to uncontrolled signaling and cancer progression. Metastatic cancer cells also show altered membrane protein diffusion.
2. Neurological disorders
In Alzheimer’s disease, altered diffusion of amyloid precursor protein may increase harmful amyloid beta production. Improper AMPA receptor diffusion affects synaptic plasticity.
3. Cardiac diseases
Abnormalities in ion channel and transporter diffusion affect cardiac conduction and can promote arrhythmias.
4. Cystic fibrosis
The cystic fibrosis transmembrane conductance regulator (CFTR) shows impaired diffusion, preventing normal chloride transport.
5. Diabetes
Diffusion of glucose transporters like GLUT4 is disrupted, contributing to insulin resistance.
6. Kidney disorders
Nephrogenic diabetes insipidus arises from improper aquaporin water channel diffusion.
7. Cholesterol disorders
Diseases like Niemann-Pick type C disease alter cholesterol diffusion and distribution.
8. Immune deficiencies
Improper diffusion of receptors like TCR and MHC alter T cell activation and cause immunodeficiencies.
9. Photoreceptor diseases
Rod and cone opsins require diffusion to renew outer segment discs. Impaired diffusion causes retinal degeneration.
10. Viral infections
Viruses can hijack membrane protein diffusion pathways and change membrane composition for entry.
In summary, aberrant membrane protein diffusion can directly contribute to pathogenesis in many human diseases. Understanding the underlying diffusion defects may reveal new therapeutic targets.
Frequently Asked Questions
What is membrane protein diffusion?
Membrane protein diffusion refers to the movement of membrane proteins laterally within the same membrane leaflet or transversely between the two bilayer leaflets. This process allows membrane proteins to localize, interact, and perform their functions properly.
Why is membrane protein diffusion important?
Membrane protein diffusion is critical for many cellular processes like cell signaling, membrane transport, organization of specialized lipid domains, and adaptive responses to stimuli. Without the ability to diffuse, membrane proteins could not migrate to their sites of action or interact with other components.
What factors affect membrane protein diffusion?
The main factors influencing membrane protein diffusion include lipid composition, temperature, direct protein-protein interactions, membrane fluidity, protein size, crowding effects, cytoskeletal interactions, and location within membrane microdomains.
How is membrane protein diffusion studied experimentally?
Common techniques for studying membrane protein diffusion include:
– Fluorescence recovery after photobleaching (FRAP).
– Single-particle tracking (SPT) of labeled proteins.
– Super-resolution microscopy methods like STED and PALM.
These approaches help quantify diffusion dynamics and visualize membrane organization.
Why is altered membrane protein diffusion linked to diseases?
Abnormal membrane protein diffusion can disrupt cell signaling, transport, and membrane domain organization. It contributes to diseases like cystic fibrosis, Alzheimer’s, viral infections, and cancer metastasis. Understanding disease-related diffusion defects may reveal new drug targets.
Conclusion
In summary, membrane protein diffusion is a vital process that enables lateral and transverse mobility within the phospholipid bilayer. This fluidity controls critical activities like cell signaling, transport, membrane organization, and adaptation.
Various techniques can probe diffusion dynamics, revealing key insights into membrane protein function in health and disease. As membrane research continues, our comprehension of the nuances regulating protein mobility will further advance.
KeyNote Points:
- Membrane proteins diffuse laterally in the lipid bilayer to perform their functions.
- Diffusion rates depend on protein size, lipid environment, crowding, interactions, membrane dimensions, compartments, modifications, and temperature.
- Fast diffusion enables cell signaling, transport, mobility, organization, and interactions.
- Slower diffusion disrupts signaling cascades, transport, cell migration, polarization, protein sorting, enzyme regulation, and organelle contacts.
- Aberrant diffusion contributes to diseases like cancer, neurodegeneration, cardiomyopathy, cystic fibrosis, diabetes, kidney disorders, cholesterol defects, immunodeficiency, and photoreceptor degeneration.
- Understanding disease diffusion defects can reveal new drug targets and treatment strategies.
- Methods to study membrane protein diffusion include fluorescence recovery after photobleaching (FRAP), single particle tracking (SPT), fluorescence correlation spectroscopy (FCS), and super-resolution microscopy.
- Computational simulations help model the complex dynamics of proteins diffusing in membrane environments.
- Overall, the lateral diffusion of membrane proteins is a fundamental biophysical process enabling membrane proteins’ dynamic organization, interactions, and function.
In summary, membrane protein diffusion is a key mechanism regulating many essential cellular activities, and disruption of diffusion can directly contribute to pathogenic states. Advancing our knowledge in this area could have important medical applications.