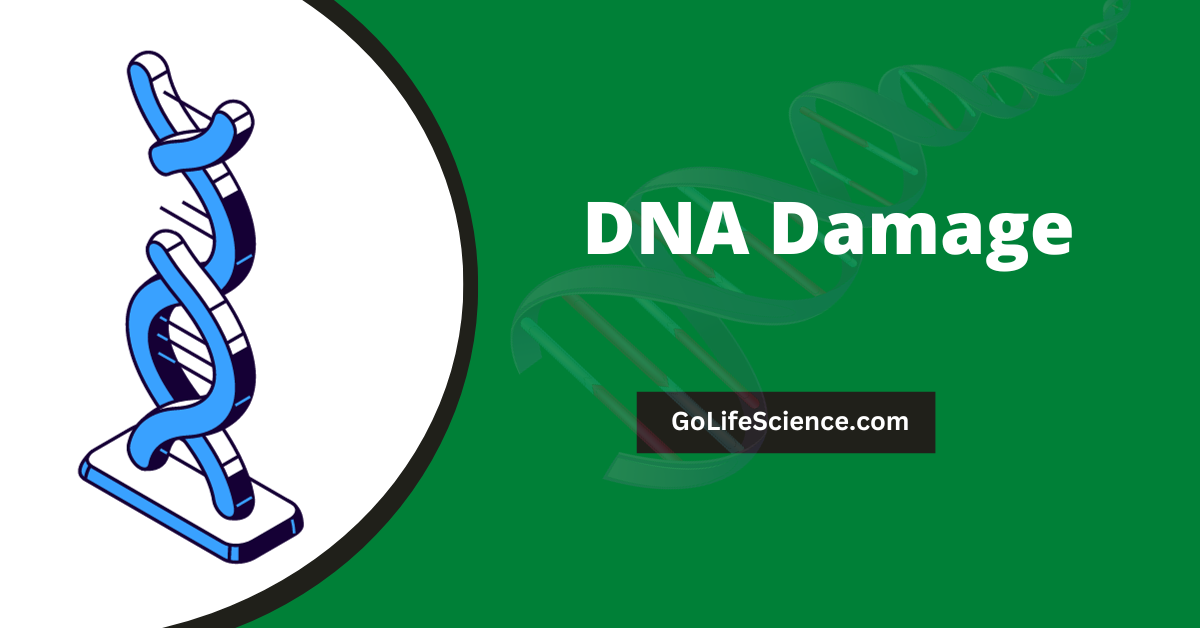
DNA, or deoxyribonucleic acid, contains the genetic blueprint essential for life. As the carrier of genetic information, DNA enables the transfer of hereditary characteristics from parents to offspring. However, DNA is susceptible to damage from various endogenous and exogenous sources. Unrepaired DNA damage can lead to genetic mutations that may result in cancer, ageing, and other diseases. Thus, understanding the mechanisms of DNA damage and repair is crucial for developing preventative and therapeutic strategies.
Watson and Crick described the double helix structure of DNA. They proposed its semi-conservative replication based on specific base pairings to explain the faithful transmission of genetic information from generation to generation mechanism to explain spontaneous mutations.
Watson and Crick pointed out that the structures of the bases in DNA are not static. Hydrogen atoms can move from one position in a purine or pyrimidine to another position, for example, an amino group to ring nitrogen. Such chemical functions are called ‘Tautomeric shifts’.
Although tautomeric shifts are rare, they may be of considerable importance in DNA metabolism since they alter the base-pairing potential of the bases. In the common stable form, adenine always pairs with thymine, and guanine always pairs with cytosine. However, these forms infrequently undergo tautomeric shifts to less stable enol and imino forms.
DNA is a molecule that encodes the genetic instructions for all living organisms’ development, function, growth and reproduction. DNA is constantly exposed to agents and factors that can alter its structure and sequence, damaging DNA.
What is DNA Damage?
DNA damage refers to structural alterations to the DNA molecule that introduce deviations from its normal double helical structure. These abnormalities interfere with DNA replication and transcription, causing mutations.
There are different types of DNA damage:
- Bulky DNA adducts: bulky chemical groups bonded to bases
- Crosslinks: bonds that improperly connect DNA strands
- Thymine dimers: abnormalities caused by UV radiation
- Oxidized bases: bases damaged by reactive oxygen species
- Double strand breaks: complete breaks in the DNA backbone
If DNA damage is not accurately repaired, it can produce permanent genetic mutations that may lead to cancer or cell death.
The Significance of DNA
DNA contains the cell’s genetic material and hereditary information. The sequence of DNA base pairs provides instructions for:
- Protein synthesis
- Cell growth and division
- Tissue and organ formation
- Organism development and function
Any changes to the DNA sequence through damage can harm these processes. Thus, DNA integrity and stability are vital for life.
The Importance of Understanding DNA Damage
Research into DNA damage is essential because:
- It causes mutations and cancer
- Accumulated DNA damage is a factor in ageing
- Many diseases are linked to defective DNA repair
- DNA is vulnerable to damage from environmental agents
- Understanding damage of DNA facilitates disease prevention and treatment
- DNA repair pathways are targets for drug development
Knowledge advances genome stability and protects genetic information.
Why DNA Damage?
They exist in these less stable tautomeric forms only for very short periods. However, suppose a base existed in its rare form when it was being replicated or incorporated into a nascent DNA chain. A mutation might result in adenine-cytosine and guanine-thymine base pairs in that case.
Examples of mismatched AC and GT base pairs can form when one of the purines or pyrimidines exists in a rare tautomeric form. Similar mismatched base pairs can be created when thymine exists in its rare enol form or adenine in its rare imino form.
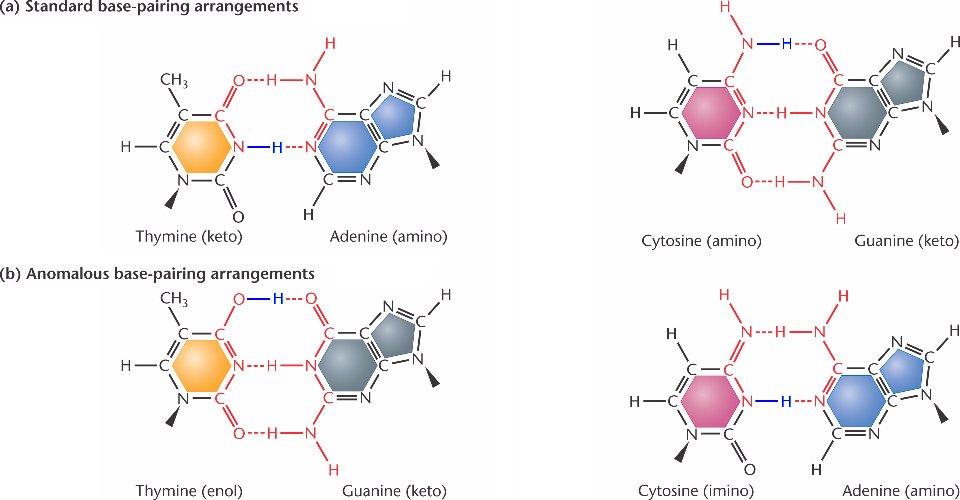
The net effect of such an event and the subsequent replication required to segregate the ‘mismatched’ is an AT, GC, or AT base pair substitution.
Mutations resulting from tautomeric shifts in the bases of DNA involve the replacement of purine and the replacement of pyrimidine in the complementary strand with the other pyrimidine. Such base-pair substitutions are called ‘Transitions’.
Base pair substitutions involving the substitution of a purine for pyrimidine and vice versa are called transversions. These are possible eight different transversions.
Base substitutions possible in DNA: Purine for Purine and Pyrimidine for Pyrimidine, Purine for Pyrimidine (dashed arrows) and vice versa (solid arrows)
The third type of point mutation involves adding or deleting one or a few base pairs. Base pair additions and deletions are called frameshift mutations because they alter the reading frame of all base pair triplets (specifying codons in mRNA and amino acids in the polypeptide gene product) in the gene detail to the mutation.
Frameshift mutation and the changes in the reading of genetic code
Suppose three residues or some multiple of three are added or subtracted. The remaining residues will still be in the correct triplet sequence for coding the intended amino acids through mRNA transcription. Therefore, the result will be forming a peptide chain with some amino acids missing or additional ones inserted.
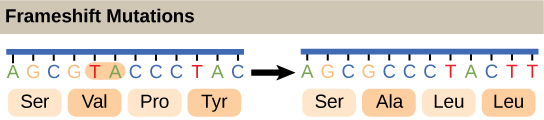
If, on the other hand, an error in replication changes one or two or some multiple not divisible by three, then the genetic code gets scrambled, and the wrong amino acid will be incorporated into the resultant polypeptide chain at nearly every position.
- Polypeptide chain conformation – Ramachandran PlotWhat is the Secondary Structure of Proteins?
- What is the role of the Tertiary Structure of Protein (Basic Guide)
All the three above-described types of point mutations, transitions, transversions, and frameshift mutations are present among spontaneously occurring mutations. A surprisingly large proportion of the spontaneous mutations studied in prokaryotes are found to be single base-pair additions and deletions rather than base-pair substitutions.
Types of DNA Damage
There are several types of DNA damage that can occur within our cells. One common type is called base oxidation, which happens when oxygen molecules react with the bases in our DNA strands, leading to changes in the genetic code. Another type is DNA strand breaks, where the backbone of the DNA molecule is severed, disrupting the integrity of the genetic material. Additionally, chemical modifications such as methylation can occur, altering the expression of genes and potentially leading to detrimental effects. Understanding these various forms of DNA damage is crucial for developing strategies to protect and repair our genetic material.
There are two major categories of DNA damage:
Spontaneous DNA Damage
It occurs naturally and inevitably over time. Sources include:
- Hydrolysis: Breakage of phosphodiester bonds
- Depurination: Removal of purine bases (adenine and guanine)
- Depyrimidination: Removal of pyrimidine bases (thymine and cytosine)
Induced DNA Damage
It results from external stressors in the cellular environment. Major causes include:
UV Radiation
- Sunlight UV exposure causes thymine dimers
- UVC rays generate reactive oxygen species that damage DNA
Chemical Agents
- Alkylating agents like mustard gas
- Intercalating agents like ethidium bromide
- Reactive oxygen species (ROS) like peroxides and free radicals
Radiation
- Ionizing radiation like X-rays, gamma rays, and radon
- Cause single and double DNA strand breaks
Sources of induced DNA damage pose genetic hazards and increase mutation rates.
Causes and Sources of DNA Damage
This damage can arise from various sources and causes. Exposure to ultraviolet (UV) radiation from the sun can result in the formation of thymine dimers, leading to DNA damage. Additionally, environmental factors such as exposure to certain chemicals or toxins, including tobacco smoke and pollutants, can introduce DNA lesions.
Internal processes, like oxidative stress and errors during DNA replication, can also cause Genetic Damage. Furthermore, certain viruses, such as human papillomavirus (HPV), can integrate their DNA into the host genome, potentially disrupting its integrity. Understanding the causes and sources of DNA damage is crucial for developing strategies to mitigate its effects.
DNA damage stems from various endogenous and exogenous sources.
a. Environmental Factors
- Radiation exposure (solar, radioactive)
- Air pollution (smog, asbestos, heavy metals)
- Certain chemicals, solvents, and pesticides
- Alcohol consumption and tobacco smoke
b. Lifestyle Factors
- Poor diet lacking micronutrients
- Obesity and lack of exercise
- Chronic psychological stress
- Insufficient sleep or sleep disturbances
c. Endogenous Factors
- Free radicals from metabolic processes
- Spontaneous hydrolytic reactions
- Replication errors
- Defects in DNA repair mechanisms
d. Replication Errors
- Incorrect nucleotide insertion
- Strand slippage
- Problems with DNA polymerases
Exposure to damaging agents combined with inadequate repair causes DNA damage to accumulate over time. This genomic instability facilitates mutagenesis and carcinogenesis.
Consequences of DNA Damage
Unrepaired DNA damage produces permanent genetic mutations that can cause:
a. Mutations and Genetic Variations
- Point mutations from single base pair changes
- Insertions, deletions, duplications, and translocations
b. Cancer Development
- Proto-oncogene activation
- Tumor suppressor gene inactivation
- Genomic instability enabling malignancy
c. Aging and Age-Related Diseases
- Cellular senescence and apoptosis
- Tissue dysfunction or atrophy
- Neurodegenerative disorders
- Cardiovascular and immune conditions
d. Cellular Dysfunction
- Impaired transcription and translation
- Mitochondrial damage and metabolic defects
- Loss of cell division and growth
DNA Repair Mechanisms
To counteract DNA damage, cells have evolved various DNA repair pathways that identify and resolve DNA lesions.
Overview of Repair Pathways
These pathways remove and replace damaged bases or nucleotides, correct mismatches, and fix double-strand DNA breaks through resection.
There are five major DNA repair mechanisms:
a. Base Excision Repair (BER)
This pathway repairs damaged bases that do not significantly distort the DNA helix, including:
- Oxidized bases
- Alkylated bases
- Deaminated bases
- Single strand breaks
BER involves damaged base recognition, removal, gap filling, and ligation.
b. Nucleotide Excision Repair (NER)
NER identifies and repairs bulky DNA lesions:
- Thymine dimers from UV radiation
- Intrastrand crosslinks
- Tobacco carcinogen adducts
It excises damaged areas in longer single-stranded segments.
c. Mismatch Repair (MMR)
MMR corrects errors in DNA replication that escape proofreading:
- Mismatched bases
- Insertion/deletion loops
- Some forms of DNA damage
MMR proteins detect distortions in the DNA helix and recruit enzymes to excise and resynthesize the defective region.
d. Homologous Recombination (HR)
HR accurately repairs double-strand breaks and interstrand DNA crosslinks. It uses the undamaged sister chromatid as a DNA synthesis and repair template.
e. Non-Homologous End Joining (NHEJ)
NHEJ directly joins broken DNA ends with no template. It is error-prone but essential for double-strand break repair in non-dividing cells.
DNA repair mechanisms are vital for maintaining genomic stability and integrity. Defects in these pathways are linked to disease susceptibility and premature ageing.
Methods for Detecting DNA Damage
Measuring DNA damage is important for genotoxicity testing and biomonitoring. Methods include:
a. Laboratory Techniques
- Gel electrophoresis detects DNA fragmentation
- PCR amplifies damaged genes
- DNA sequencing maps genetic mutations
- Comet assay visualizes DNA strand breaks
b. Biomarkers and Assays
- Immunoassays quantify damaged nucleosides
- H2AX measures double-strand breaks
- Micronucleus test evaluates chromosomal damage
- Ames test determines mutagenicity
c. Clinical Applications
- DNA damage assays predict cancer risk
- Quantifying UV-induced lesions aids in melanoma diagnosis
- Measuring oxidative DNA damage indicates diseases
- Monitoring DNA repair activity guides treatments
Developments in analytical tools continue to enhance DNA damage detection, analysis, and applications.
DNA Damage and Disease
DNA damage is implicated in the pathogenesis of many diseases:
a. DNA Damage in Cancer
- Mutation of oncogenes activates proliferation
- Loss of tumour suppressors enables malignancy
- Genomic instability facilitates the progression
- Alterations in DNA repair genes increase the risk
b. DNA Damage in Neurodegenerative Diseases
- Oxidative lesions in mitochondrial and nuclear DNA
- Accumulation of DNA damage overwhelms repair capacity
- Neuronal death and brain atrophy
c. DNA Damage in Autoimmune Diseases
- Release of DNA damage response proteins triggers inflammation
- Loss of DNA repair induces cell death and tissue damage
- Environmental triggers like radiation and chemicals
Additionally, DNA repair defects cause disease vulnerability. Patients with inherited DNA repair disorders suffer from cancers, neurodegeneration, immunodeficiency, and premature ageing syndromes.
Strategies for DNA Damage Prevention and Repair
Methods to reduce and treat DNA damage include:
a. Lifestyle Changes
- Avoid UV exposure, chemicals, toxins
- Adopt cancer-preventative behaviours
- Consume antioxidants like vitamins C and E
- Manage psychological stress
- Exercise regularly
b. Antioxidants and Protective Agents
- Nutraceuticals like curcumin and EGCG
- Carotenoids, flavonoids, and polyphenols
- Enzyme cofactors like zinc and magnesium
- Phytochemicals that boost endogenous antioxidants
c. Emerging Therapies
- Small molecules to modulate DNA repair pathways
- Nanoparticles to deliver DNA repair proteins
- Genome editing tools to correct mutations
- Stem cell therapies to replace damaged cells
d. Radiation and Chemoprotectants
- Amifostine protects against radiotherapy toxicity
- Dexrazoxane guards against anthracycline damage
- Mesna mitigates ifosfamide chemotherapy side effects
A multifaceted strategy is required to minimize DNA damage and mutations for disease prevention.
Future Directions in DNA Damage Research
Advancing DNA damage and repair research is critical to expand our knowledge and capabilities. Future progress involves:
a. Advances in Technology and Tools
- High-throughput sequencing to map mutational profiles
- Novel imaging techniques like near-infrared spectroscopy
- Microfluidics and organ-on-a-chip models
- Artificial intelligence for data analysis
b. Potential Breakthroughs and Discoveries
- Defining roles of non-coding RNAs in DNA repair
- Understanding dysfunction in age-related disorders
- Expanding insights into DNA repair mechanisms
- Harnessing DNA damage response for cancer therapy
- Developing personalized treatments based on mutations
Ongoing research will uncover new DNA damage and repair biological aspects for better health outcomes.
Frequently Asked Questions on DNA Damage
What is DNA damage?
DNA damage refers to abnormalities and lesions in the DNA molecule resulting from exposure to endogenous and environmental agents. These alterations interfere with DNA replication and transcription, causing mutations.
What causes DNA damage?
Environmental factors like radiation, chemicals, toxins, and lifestyle behaviours can cause DNA damage. It also arises from endogenous sources like reactive oxygen species, hydrolytic reactions, and DNA replication errors.
What are the consequences of DNA damage?
Unrepaired DNA damage leads to genetic mutations and instability. It can result in diseases like cancer, neurodegeneration, immunodeficiency disorders, and premature ageing. Even normal ageing arises in part from lifelong accumulation of DNA damage.
How is DNA damage repaired in cells?
Cells have multiple DNA repair pathways identifying and resolving DNA damage, including base excision repair, nucleotide excision repair, mismatch repair, homologous recombination, and non-homologous end joining.
How can DNA damage be prevented or limited?
Strategies like lifestyle changes, antioxidants, dietary phytochemicals, and emerging therapies like genome editing tools can help minimize DNA damage. Avoiding exposure to radiation, chemicals, and toxins also reduces DNA damage.
Conclusion
DNA integrity is vital for life. Various endogenous and exogenous agents continuously damage DNA. Unrepaired lesions lead to deleterious genetic mutations, causing cancer, neurodegeneration, immunodeficiency, premature ageing, and cell death. Cells have evolved multiple repair pathways to resolve different forms of DNA damage and maintain genomic stability.
Defects in DNA repair mechanisms are linked to human diseases, underscoring the necessity of these processes for health. Further research into DNA damage and repair will expand our understanding of associated disorders, ageing, and potential treatments. Novel technologies will enable high-throughput DNA damage analysis, propelling diagnostic and therapeutic capabilities.
A comprehensive approach involving lifestyle changes, protective agents, emerging therapies, and early detection strategies is imperative to reduce DNA damage for optimal well-being. As our knowledge grows, we edge closer to elucidating DNA damage intricacies for mitigating disease susceptibility.