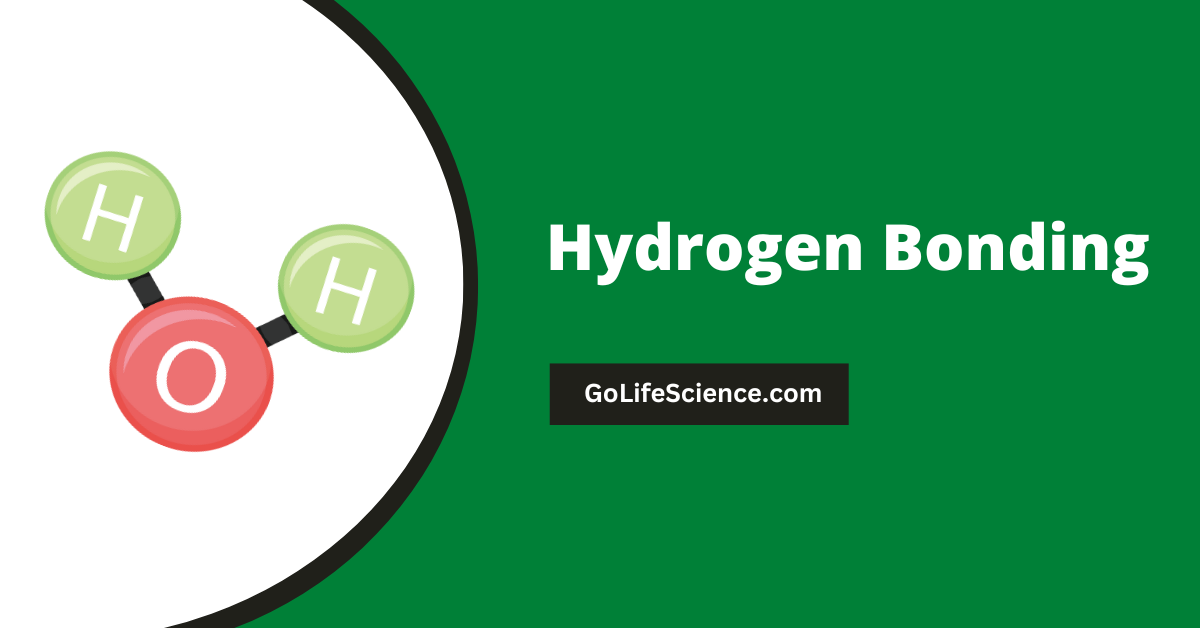
Hydrogen bonding is one of the most fundamental and fascinating phenomena in chemistry and biology. It plays a crucial role in determining the structure, properties, and functions of many biological molecules, including water, proteins, and DNA. Despite being a relatively weak interaction compared to covalent or ionic bonds, hydrogen bonding is essential for life as we know it. This article delves into the science behind hydrogen bonding, its significance in life sciences, and its applications in various fields.
Table of Contents
What is Hydrogen Bonding?
Hydrogen bonding is a type of dipole-dipole interaction that occurs when a hydrogen atom covalently bonded to a highly electronegative atom (such as nitrogen, oxygen, or fluorine) experiences an attractive force with another electronegative atom in close proximity. This interaction is represented as X-H···Y, where X and Y are electronegative atoms, and the dotted line represents the hydrogen bond.
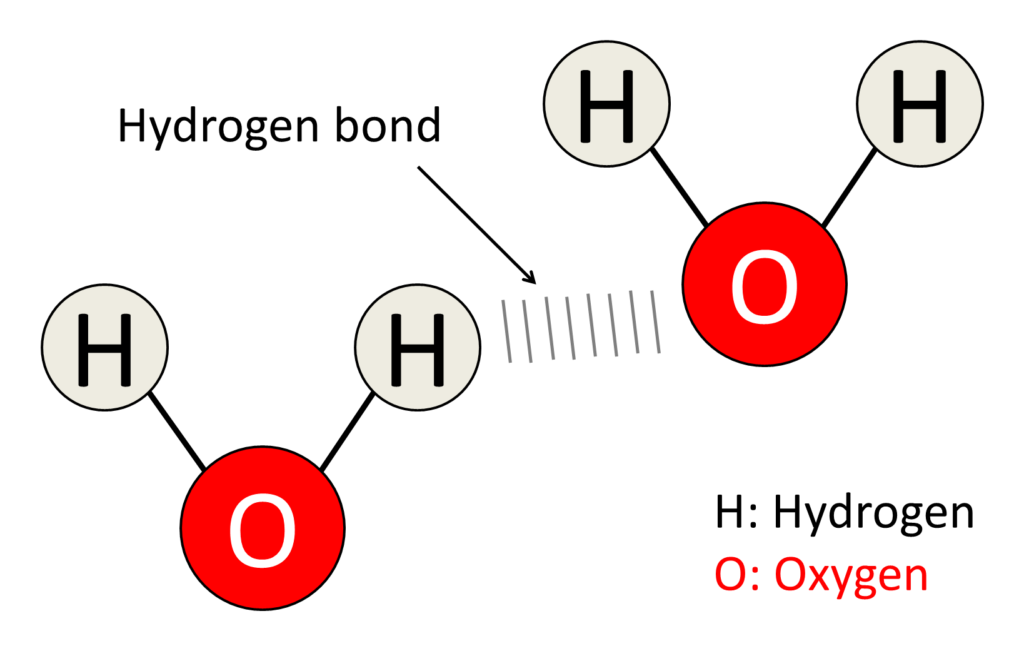
Characteristics of Hydrogen Bonds
- Strength: Hydrogen bonds are weaker than covalent and ionic bonds but stronger than van der Waals forces. Typically, they have energies ranging from 4 to 40 kJ/mol.
- Directionality: Hydrogen bonds are highly directional, with the hydrogen atom aligning itself along the line connecting the two electronegative atoms.
- Distance: The optimal distance between the hydrogen atom and the acceptor atom (Y) is usually between 2.7 to 3.1 Å.
Types of Hydrogen Bonds
- Intermolecular Hydrogen Bonds: These occur between molecules. For example, hydrogen bonds between water molecules contribute to water’s high boiling point and surface tension.
- Intramolecular Hydrogen Bonds: These occur within a single molecule. An example is the hydrogen bonding in proteins and nucleic acids, which helps stabilize their structures.
The Role of Hydrogen Bonding in Water
Water is often referred to as the “universal solvent,” and its unique properties are largely due to hydrogen bonding.
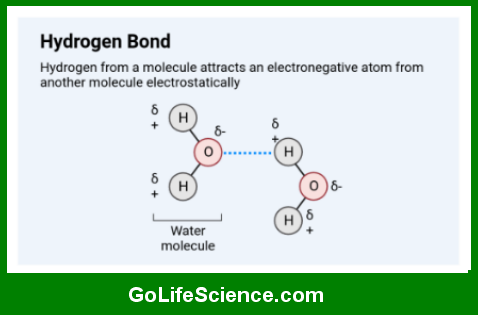
- High Boiling and Melting Points: Hydrogen bonds between water molecules require more energy to break, resulting in higher boiling and melting points compared to similar-sized molecules.
- High Specific Heat: Water can absorb a lot of heat before its temperature rises, making it an excellent temperature buffer.
- Surface Tension: Hydrogen bonds create a strong cohesive force at the surface of water, allowing insects to walk on water and droplets to form.
- Density Anomaly: Water is densest at 4°C, and ice floats on water due to the hydrogen-bonded lattice structure, which is less dense than liquid water.
Biological Implications
The properties of water are crucial for life. They enable:
- Temperature Regulation: Organisms can maintain stable internal temperatures.
- Solvent Properties: Water dissolves a wide range of substances, facilitating biochemical reactions.
- Transport Medium: Water transports nutrients and waste products in biological systems.
Hydrogen Bonding in Biological Molecules
Hydrogen bonding is a critical force in the structure, stability, and function of biological molecules. From the intricate folding of proteins to the precise pairing of DNA strands, hydrogen bonds act as the molecular glue that holds life together. This section delves deeper into the role of hydrogen bonding in proteins, nucleic acids, and carbohydrates, highlighting its significance in maintaining the integrity and functionality of these essential biomolecules.
Hydrogen Bonding in Proteins
Proteins are the workhorses of the cell, performing a vast array of functions, including catalyzing biochemical reactions, providing structural support, and facilitating cellular communication. The structure and function of proteins are intimately tied to hydrogen bonding.
1. Secondary Structure: Alpha-Helices and Beta-Sheets
The secondary structure of proteins refers to localized folding patterns stabilized primarily by hydrogen bonds. The two most common secondary structures are alpha helices and beta sheets.
- Alpha-Helices: In an alpha-helix, the polypeptide chain coils into a right-handed spiral. Hydrogen bonds form between the carbonyl oxygen (C=O) of one amino acid and the amide hydrogen (N-H) of another amino acid four residues away. This pattern creates a stable, rod-like structure. Alpha helices are common in structural proteins like keratin (found in hair and nails) and in the transmembrane domains of membrane proteins.
- Beta-Sheets: Beta-sheets consist of extended polypeptide strands (beta-strands) that align side by side, connected by hydrogen bonds between the carbonyl and amide groups of adjacent strands. Beta-sheets can be parallel (strands run in the same direction) or antiparallel (strands run in opposite directions). These structures are found in proteins like silk fibroin and immunoglobulins (antibodies).
2. Tertiary Structure: Protein Folding
The tertiary structure of a protein refers to its overall three-dimensional shape, which is stabilized by various interactions, including hydrogen bonds, ionic bonds, hydrophobic interactions, and disulfide bridges. Hydrogen bonds play a key role in stabilizing the folded conformation of proteins by forming between side chains (R groups) and backbone atoms.
For example:
- Globular Proteins: In enzymes like lysozyme, hydrogen bonds help maintain the compact, globular structure necessary for catalytic activity.
- Fibrous Proteins: In collagen, hydrogen bonds between hydroxyproline residues contribute to the triple-helix structure, providing tensile strength to connective tissues.
3. Quaternary Structure: Protein Assembly
Many proteins consist of multiple polypeptide chains (subunits) that assemble into a functional complex. Hydrogen bonds between subunits help stabilize the quaternary structure. For instance:
- Hemoglobin: The four subunits of hemoglobin are held together by hydrogen bonds, enabling cooperative binding of oxygen.
- Antibodies: The Y-shaped structure of antibodies is stabilized by hydrogen bonds between heavy and light chains.
4. Protein Function and Dynamics
Hydrogen bonds are not only structural but also functional. They play a role in:
- Enzyme Catalysis: Hydrogen bonds stabilize transition states and intermediates during enzymatic reactions.
- Ligand Binding: Hydrogen bonds between proteins and ligands (e.g., substrates, inhibitors) provide specificity and strength to interactions.
Hydrogen Bonding in Nucleic Acids
Nucleic acids, including DNA and RNA, are the carriers of genetic information. Hydrogen bonding is essential for their structure, stability, and function.
1. DNA Double Helix
The iconic double-helix structure of DNA is stabilized by hydrogen bonds between complementary base pairs.
- Base Pairing: Adenine (A) forms two hydrogen bonds with thymine (T), and guanine (G) forms three hydrogen bonds with cytosine (C). This complementary base pairing ensures the accurate replication and transcription of genetic information.
- Helix Stability: Hydrogen bonds, along with base stacking interactions, stabilize the double helix, allowing DNA to withstand thermal fluctuations and mechanical stress.
2. DNA Replication and Transcription
Hydrogen bonds play a dynamic role in DNA replication and transcription:
- Replication: During DNA replication, hydrogen bonds between base pairs are temporarily broken by helicase enzymes, allowing the DNA strands to separate and serve as templates for new strands.
- Transcription: During transcription, hydrogen bonds between DNA and RNA nucleotides facilitate the synthesis of messenger RNA (mRNA).
3. RNA Structure and Function
RNA molecules, such as mRNA, transfer RNA (tRNA), and ribosomal RNA (rRNA), rely on hydrogen bonding for their structure and function.
- Secondary Structure: Hydrogen bonds stabilize hairpin loops, stem-loops, and other secondary structures in RNA. For example, tRNA adopts a cloverleaf structure stabilized by hydrogen bonds.
- Tertiary Structure: In ribozymes (catalytic RNA molecules), hydrogen bonds help maintain the three-dimensional structure necessary for catalytic activity.
- RNA-DNA Hybrids: During transcription, hydrogen bonds form between the nascent RNA strand and the DNA template, ensuring accurate RNA synthesis.
Hydrogen Bonding in Carbohydrates
Carbohydrates, including sugars and polysaccharides, are essential for energy storage, structural support, and cell signaling. Hydrogen bonding plays a key role in their structure and function.
1. Monosaccharides and Disaccharides
In simple sugars, hydrogen bonds form between hydroxyl (-OH) groups, influencing their solubility and interactions with other molecules.
- Solubility: The ability of sugars like glucose and sucrose to form hydrogen bonds with water makes them highly soluble, facilitating their transport in biological systems.
- Crystal Formation: In solid states, hydrogen bonds between sugar molecules contribute to crystal formation, as seen in table sugar (sucrose).
2. Polysaccharides
Polysaccharides are long chains of monosaccharides linked by glycosidic bonds. Hydrogen bonding between chains contributes to their structural properties.
- Cellulose: In plant cell walls, cellulose chains are held together by hydrogen bonds, forming microfibrils that provide rigidity and strength. The extensive hydrogen bonding network makes cellulose insoluble in water.
- Chitin: In the exoskeletons of arthropods and the cell walls of fungi, chitin chains are stabilized by hydrogen bonds, providing structural integrity.
- Glycogen and Starch: These energy storage polysaccharides have branched structures where hydrogen bonds help maintain their compact, globular forms.
3. Carbohydrate-Protein Interactions
Hydrogen bonds are crucial in carbohydrate-protein interactions, which play roles in cell signaling, immune response, and pathogen recognition.
- Glycoproteins: Proteins with attached carbohydrate chains (glycans) often rely on hydrogen bonds for their stability and function. For example, the glycoproteins on cell surfaces are involved in cell-cell recognition and communication.
- Lectin-Carbohydrate Binding: Lectins are proteins that bind specifically to carbohydrates through hydrogen bonds, mediating processes like immune response and pathogen attachment.
Hydrogen Bonding in Lipids
While lipids are primarily nonpolar, hydrogen bonding can occur in certain lipid molecules, particularly those with polar head groups.
1. Phospholipids
Phospholipids are the main components of cell membranes. Their polar head groups (e.g., phosphate and choline) can form hydrogen bonds with water and other polar molecules.
- Membrane Fluidity: Hydrogen bonds between phospholipid head groups and water molecules contribute to the fluidity and stability of cell membranes.
- Lipid Bilayer Formation: The amphipathic nature of phospholipids, driven in part by hydrogen bonding, allows them to spontaneously form bilayers in aqueous environments.
2. Sphingolipids and Glycolipids
Sphingolipids and glycolipids, which contain carbohydrate groups, rely on hydrogen bonding for their structural and functional roles.
- Cell Signaling: Hydrogen bonds between glycolipids and proteins are involved in cell signaling and recognition processes.
- Membrane Microdomains: Hydrogen bonding contributes to the formation of lipid rafts, specialized microdomains in cell membranes that play roles in signaling and trafficking.
Hydrogen bonding is a fundamental force that shapes the structure and function of biological molecules. In proteins, it stabilizes secondary, tertiary, and quaternary structures, enabling their diverse functions. In nucleic acids, it ensures the accurate storage and transmission of genetic information. In carbohydrates, it contributes to structural integrity and molecular interactions. Even in lipids, hydrogen bonding plays a role in membrane dynamics and signaling.
Hydrogen Bonding in Drug Design
Molecular Recognition
Hydrogen bonding is critical in the interaction between drugs and their target molecules.
- Receptor-Ligand Interactions: Drugs often bind to their targets through hydrogen bonds, which provide specificity and strength to the interaction.
- Enzyme Inhibition: Many drugs inhibit enzymes by forming hydrogen bonds with active site residues, preventing the enzyme from functioning.
Solubility and Bioavailability
Hydrogen bonding affects the solubility and bioavailability of drugs.
- Solubility: Drugs that can form hydrogen bonds with water are more soluble, which is crucial for their absorption and distribution in the body.
- Bioavailability: The ability of a drug to form hydrogen bonds with biological membranes can influence its permeability and bioavailability.
Hydrogen Bonding in Material Science
Polymers
Hydrogen bonding is used to design polymers with specific properties.
- Self-Healing Materials: Some polymers can self-heal due to reversible hydrogen bonds that reform after damage.
- Stimuli-Responsive Materials: Polymers that change properties in response to stimuli (like temperature or pH) often rely on hydrogen bonding.
Supramolecular Chemistry
Hydrogen bonding is a key interaction in supramolecular chemistry, which studies complex molecular assemblies.
- Molecular Recognition: Hydrogen bonds are used to create molecular recognition sites in supramolecular structures.
- Self-Assembly: Hydrogen bonding drives the self-assembly of molecules into larger, functional structures.
Hydrogen Bonding in Environmental Science
Water Purification
Hydrogen bonding is exploited in water purification technologies.
- Reverse Osmosis: Membranes used in reverse osmosis rely on hydrogen bonding to selectively allow water molecules to pass while blocking contaminants.
- Adsorption: Materials like activated carbon use hydrogen bonding to adsorb pollutants from water.
Climate Science
Hydrogen bonding in water vapor affects climate dynamics.
- Greenhouse Effect: Water vapor is a significant greenhouse gas, and its ability to form hydrogen bonds influences its radiative properties.
- Cloud Formation: Hydrogen bonding is involved in the nucleation of water droplets in clouds, impacting weather patterns.
Conclusion
Hydrogen bonding is a subtle yet powerful force that underpins many of the processes essential to life and technology. From the unique properties of water to the intricate structures of proteins and DNA, hydrogen bonding is a cornerstone of biochemistry and molecular biology.
Its applications in drug design, material science, and environmental technology further highlight its importance. Understanding hydrogen bonding not only deepens our appreciation of the natural world but also opens up new avenues for scientific and technological innovation.
As we continue to explore the complexities of life at the molecular level, hydrogen bonding will undoubtedly remain a key area of study, offering insights and solutions to some of the most pressing challenges in science and medicine. Whether you’re a student, researcher, or simply a curious mind, the world of hydrogen bonding is a fascinating realm to explore.
Frequently Asked Questions (FAQs)
What is a hydrogen bond, and why is it important in biology?
A hydrogen bond is a weak attraction between a hydrogen atom and an electronegative atom like oxygen or nitrogen. It’s crucial in biology because it stabilizes the structure of proteins, DNA, and other biomolecules, enabling life processes.
How do hydrogen bonds help DNA stay together?
Hydrogen bonds form between complementary base pairs (A-T and G-C) in DNA, holding the two strands of the double helix together while allowing them to separate for replication and transcription.
Why does water have such unique properties?
Water’s unique properties, like high boiling point and surface tension, come from hydrogen bonds between its molecules, making it essential for life.
Can hydrogen bonds break and reform?
Yes! Hydrogen bonds are dynamic—they can break and reform easily, which is why they’re perfect for processes like DNA replication and protein folding.
How do hydrogen bonds affect protein shape?
Hydrogen bonds stabilize protein structures like alpha-helices and beta-sheets, ensuring proteins fold into their functional shapes.
What role do hydrogen bonds play in enzymes?
Hydrogen bonds help enzymes maintain their shape and stabilize interactions with substrates, making them efficient biological catalysts.
Why is cellulose so strong?
Cellulose chains are held together by a network of hydrogen bonds, creating a rigid structure that provides strength to plant cell walls.
How do hydrogen bonds help drugs work?
Drugs often bind to their targets through hydrogen bonds, ensuring specificity and strength in their interactions, which is key to their effectiveness.
Do hydrogen bonds exist in RNA?
Yes! Hydrogen bonds stabilize RNA’s secondary and tertiary structures, like hairpin loops, which are essential for its function in protein synthesis.
Can hydrogen bonding be used in technology?
Absolutely! Hydrogen bonding is used in designing self-healing materials, water purification systems, and even drug delivery systems.